Original Article
|
|
| |
Year : 2018 | Volume
: 6
| Issue : 3 |
Page : 43-49 |
|
|
Antioxidant and hepatoprotective potential of Hydnocarpus laurifolia: an in vitro and in vivo evaluation
Kumarapalayam R. Yuvaraja, Arockiasamy Santhiagu, Shabbeer Jasemine
Correspondence Address:Department of Pharmaceutical Chemistry, KMCH College of Pharmacy, Coimbatore, Tamil Nadu, India, Bioprocess Lab, School of Biotechnology, National Institute of Technology, Calicut, Kerala, India, Mary Labs, Manassery, Calicut, Kerala, India
Source of Support: Nil,
Conflict of Interest: None declared
 |
Check
|
DOI: 10.4103/2231-4040.197331
Seeds of Hydnocarpus laurifolia (HL) have been used to cure hepatitis by folk medicinal practitioners in the Wayanad hills of Western Ghats of India. The present study was undertaken to evaluate the antioxidant and hepatoprotective activities of the chloroform extract of HL (CHL) using in vitro and in vivo models. The total phenolic and total flavonoid contents of CHL were determined by standard methods. In vitro antioxidant effect of CHL was ascertained by total reduction capability, inhibition of lipid peroxidation and the ability of CHL to scavenge 2,2-diphenyl-1-picrylhydrazyl (DPPH), superoxide anion and hydroxyl free radicals using standard protocols. In vivo antioxidant and hepatoprotective potential of CHL (100 mg/kg; 200 mg/kg) was studied by paracetamol (PCM)-induced hepatotoxicity in Wistar albino rats. CHL exhibited strong in vitro antioxidant activity as demonstrated by the reduction of Fe3+ to Fe2+ (EC50, 320 μg/ml), scavenging of DPPH (IC50, 320 μg/ml), superoxide anion (IC50, 280 μg/ml), hydroxyl (IC50, 290 μg/ml) radicals, and by the inhibition of lipid peroxidation (IC50, 230 μg/ml). CHL was effective in normalizing the serum levels of hepatic enzymes glutamate-oxaloacetate transaminase, glutamate-pyruvate transaminase, alkaline phosphatase, total protein, and bilirubin in PCM intoxicated rats. The extract significantly enhanced the levels of hepatic antioxidant enzymes superoxide dismutase, catalase, glutathione peroxidase, and glutathione and reduced the thiobarbituric acid reactive substances level in PCM intoxicated rat liver. Histopathological studies of experimental animal liver tissues also confirmed the hepatoprotection exerted by CHL. The observations of this study concluded that CHL possesses significant in vitro and in vivo antioxidant and hepatoprotective effects.
Keywords: Antioxidant, free radical scavenging, hepatoprotective, paracetamol, hydnocarpus laurifolia
How to cite this article:
Yuvaraja KR, Santhiagu A, Jasemine S. Antioxidant and hepatoprotective potential of Hydnocarpus laurifolia: An in vitro and in vivo evaluation. J Pharm BioSci 2018;6(3):43-49.
|
Introduction
Hydnocarpus laurifolia (HL) belonging to the family of Flacourtiaceae is distributed in the tropical forests along the Western Ghats (South India). Its seeds yield oil, known as chaulmoogra oil. It contains glycerides of cyclopentenyl fatty acids such as hydnocarpic acid, chaulmoogric acid, gorlic acid, oleic acid, and palmitic acid. Seed oil is highly esteemed as a cure for leprosy. It is also used for many other skin diseases.[1] Seeds and oil of this plant are acrid, bitter, thermogenic, purgative, vermifuge, hematinic, and tonic. They are used in the treatment of leprosy, skin diseases, leukoderma, dermatitis, bronchopathy, eczema, tubercular laryngitis, verminosis, wounds, ulcers, and also as a fish poison.[2,3] Liver is a vital organ involved in metabolism of foreign compounds entering the body and regulates the internal chemical environment. Human exposure to toxic substances causes liver toxicity inflicted by hepatotoxic agents. Reactive oxygen species (ROS) such as superoxide anion, hydroxyl radical (OH), and hydrogen peroxide have close association with oxidative stress. Overproduction of ROS can exacerbate the quantum of oxidative stress leading to unifying mechanism of injury that occurs in developments of clinical disease processes such as heart disease, liver damage, cancer, diabetes, and aging.[4-8] Antioxidant enzymes such as superoxide dismutase (SOD), catalase (CAT), and glutathione peroxidase (GPx) play a significant role in the prevention of tissue damage by oxidative stress through inhibition of free radicals. Conventional drugs used to cure liver toxicity are inefficient, and there is an imperative need to find drugs that possess not only improved liver protection but also significant antioxidant property. Even though HL is a main constituent of ethnomedicinal formulations used in Indian traditional medicine, there is no systemic investigation done on this plant to support the traditional claim. The present investigation was undertaken to assess the antioxidant and hepatoprotective effects of HL. The versatile nature of HL as a medicinal plant encouraged us to undertake the present study.
Materials and Methods
Chemicals and reagents
Thiobarbituric acid (TBA), trichloroacetic acid (TCA), 2,2-diphenyl- 1-picrylhydrazyl (DPPH), ethylenediaminetetraacetic acid (EDTA), quercetin, rutin, butylated hydroxyl anisole (BHA), and gallic acid, were purchased from Sigma-Aldrich (St Louis, USA). Paracetamol (PCM) was obtained from Merck Chemical Co., and Silymarin (SLY) was procured from Micro Labs Ltd. All other chemicals and solvents used were of analytical grade. Diagnostic kits for the estimation of liver function test were purchased from Ranbaxy Diagnostics Ltd., New Delhi, India.
Plant material
Seeds of HL were collected from mountainous regions of Wayanad, Kerala, India during October–November 2011 and were authenticated by a botanist Dr. V. Chelladurai. A voucher specimen of the plant was deposited at the herbarium of School of Biotechnology, National Institute of Technology Calicut (SBTH11.14).
Preparation of plant extract
The seeds of HL (2 kg) was dried, milled, and continuously extracted with methanol steam distillation in Soxhlet apparatus for 48 h. The extract was evaporated until the solvent was completely removed using a rotary vacuum evaporator and dried (240 g).
Estimation of total phenolic content
The extract was analyzed for total phenolic content (TPC) by the method described by Singleton et al.[9] Chloroform extract of HL (CHL) (0.5 ml, 1 mg/ml) was mixed with 1 ml of Folin–Ciocalteu reagent (10% v/v) followed by the addition of 5 ml of saturated sodium carbonate solution (7.5% w/v). The mixture was incubated at 30°C for 2 h. The absorbance of the solution was measured in an ultraviolet–Visible spectrophotometer (PG Instruments, UK) at 765 nm. The results were expressed as mg/g of gallic acid equivalent (GAE).
Estimation total flavonoid content
Aluminum chloride colorimetric method[10] was used to estimate the flavonoid content of the plant extract. CHL (1 mg/ml) was mixed with 1 ml of 10% (w/v) aluminum chloride, 2 ml of 1 M potassium acetate, and 2 ml distilled water. The mixture was allowed to stand at room temperature for 30 min, and the absorbance of the reaction mixture was measured at 450 nm. A standard graph using rutin (5–50 μg/ml) was drawn, and the total flavonoid content was estimated on the basis of the standard graph.
In vitro antioxidant evaluation
Total reduction capability
Total reduction capability of CHL was estimated using the method of Oyaizu.[11] CHL at different concentration (50–500 μg/ml) in 1 ml of distilled water was mixed with 2.5 ml of 0.2 M phosphate buffer (pH 6.6) and 2.5 ml of 1% (w/v) potassium ferricyanide. The mixture was incubated for 20 min at 50°C, and the reaction was terminated by the addition of 2.5 ml of 10% (w/v) TCA. Then, the mixture was centrifuged for 10 min at 5000 rpm. The supernatant (2.5 ml) was mixed with 2.5 ml of distilled water and 0.5 ml of freshly prepared 0.1 % FeCl3, and the absorbance was measured at 700 nm by a spectrophotometer (PG Instruments, UK). BHA was used as a standard, and all the experiments were done in triplicate and averaged. The reductive ability of the tested samples increased with the absorbance, and the concentration at which absorbance was 0.5 was taken as EC50.
Lipid peroxidation inhibition activity
The ability of the extract to inhibit lipid peroxidation was evaluated by estimating the thiobarbituric acid reactive substances (TBARS) using the method of Ohkawa et al. (1979) with modifications.[12,13] Freshly excised rat liver was homogenized to get 25% liver homogenate in cold phosphate-saline buffer (pH 7.4) and filtered to get a clear homogenate. The reaction mixture contained 3 ml of liver homogenate, different concentrations of CHL (50–500 μg/ml) and the lipid peroxidation was initiated by adding 100 μl of 15 mM ferrous sulfate. The reaction mixture was incubated for 30 min, and 100 μl of this mixture was mixed with 1.5 ml of 10% TCA for 10 min. Then, the mixture was centrifuged, and the supernatant was mixed with 1.5 ml of 0.67% (w/v) TBA in acetic acid. The resulting mixture was heated in a boiling water bath for 30 min, cooled and the absorbance was measured at 535 nm. The extract was replaced with buffer and BHA for control and standard, respectively. The percent inhibition of lipid peroxidation was calculated as:
Inhibition (%) = (Absorbance of control at 535 nm − Absorbance of the sample at 535 nm)/Absorbance of control at 535 nm × 100.
DPPH radical scavenging activity
The stable DPPH radical scavenging activity of CHL was appraised using the method described by Blois.[14] About 1 ml of the extract (50–500 μg/ml) was mixed with 1 ml of DPPH in methanol solution (0.1 mM) and incubated at 37°C for 30 min. The absorbance of each reaction mixtures was measured at 517 nm using a spectrophotometer. BHA was used as standard, and all the experiments were done in triplicates. DPPH scavenging ability was calculated as:
DPPH radical scavenging activity (%) = (1- Absorbance of the sample at 517 nm/Absorbance of control at 517 nm) × 100.
Superoxide anion radical scavenging activity assay
Superoxide anion radical scavenging ability of CHL was estimated by the nitroblue tetrazolium (NBT) reduction method.[15] The reaction mixture contained 1 ml of CHL (50–500 μg/ml), 2 ml of 468 μM NADH solution in 100 mM phosphate buffer (pH 7.4), and 2 ml of 156 μM NBT in 100 mM phosphate buffer (pH 7.4) and the reaction was initiated by the addition of 1 ml phenazine methosulfate (PMS) solution (60 μM PMS in 100 mM phosphate buffer, pH 7.4). The reaction mixture was incubated at 25°C for 5 min, and the absorbance was recorded at 560 nm using blank samples, containing all the reagents except PMS. The extract was replaced with solvent and standard (BHA) for negative control and positive control, respectively. All the experiments were done in triplicate, and the superoxide radical scavenging ability was calculated by the following equation:
Inhibition (%) = (1- Absorbance of the sample at 560 nm/Absorbance of control at 560 nm) × 100.
OH scavenging activity
The assay was based on the method described by Aruoma and Halliwell, 1987.[16] The reaction mixture contained 1 ml of CHL (50–500 μg/ml), 1 ml of 10 mM 2-deoxyribose, 1 ml of 10 mM FeSO4-EDTA, 1.5 ml of phosphate buffer (0.2 M, pH 7.0), and of 1 ml of water. The reaction was initiated by adding 1 ml of 10 mM H2O2 and incubated at 37°C for 4 h and stopped by the addition of 1.5 ml of 2.8% TCA and 2 ml of 1% (w/v) thiobarbituric acid in 50 mM NaOH. The solution was boiled for 10 min and cooled. The absorbance of the solution was measured at 520 nm and BHA was used as control. OH scavenging effect was calculated as:
Hydroxyl radical scavenging activity (%) = (1- Absorbance of the sample at 520 nm/Absorbance of control at 520 nm) × 100.
Pharmacological studies
Experimental animals
Male albino Wistar rats (150–200 g) used in the present study were procured from Sri Venkateshwara Enterprises, Bengaluru, India. All the animals were kept under standard laboratory conditions (temperature 25 ± 2°C and 12 h light/12 h dark cycle) and acclimatized for 1 week before commencement of the experiment. They were allowed to have free access to standard pellet food (National Institute of Nutrition, Hyderabad, India) and water ad libitum. The protocol used in the present study was approved by the Animal Ethics Committee of KMCH College of pharmacy in accordance with the guidelines for the care and use of laboratory animals set by CPCSEA.
Behavioral and toxicological studies
Six groups of each 10 Wistar albino rats of uniform weight were made and administrated with graded doses of CHL (100, 200, 400, 800, 1600, and 2000 mg/kg) and one group received 1% (w/v) carboxymethyl cellulose (control group). The entire group of animals was observed continuously for 1 h for any untoward behavioral changes and death for 2 h and then periodically for the next 12 h and then again at 24 h after CHL administration.
PCM-induced hepatotoxicity
Male Wistar albino rats were divided into six groups of 6 animals each and received orally the following treatment for 7 days. Group I (normal control) received 1% sodium carboxymethyl cellulose (1 ml/kg); Group II (PCM control) was given PCM (1 g/kg). Groups III and IV received both SLY (50 and 100 mg/kg, respectively) and PCM (1 g/kg). Groups V and VI were administrated with 100 and 200 mg/kg of CHL extract, respectively, and PCM (1 g/kg), respectively. The PCM administration was carried out 1 h after the administration of standard/test drug for Groups III–VI. All the treatments were done orally by means of a gastric tube and continued for 7 days. On the 8th day of the experiment, all the animals were anesthetized, and blood samples were collected from retro-orbital plexus. The blood samples were allowed to clot for 1 h at room temperature, and the serum was separated by centrifugation at 2500 rpm at 4°C for 15 min. Then, the rats were sacrificed, and the liver was excised, washed and perfused with ice-cold normal saline. The perfused liver samples were then homogenized in an ice bath using homogenizer in chilled 10 mM Tris–HCl (pH 7.4) to yield 10% (w/v) liver homogenate for the estimation of biochemical parameters.
Estimation of in vivo hepatoprotective markers
Serum levels of hepatic enzymes glutamate-oxaloacetate transaminase (GOT) and glutamate-pyruvate transaminase (GPT), alkaline phosphatase (ALP), total bilirubin (BRN), and total protein (TP) were measured by the methods described by Reitman and Frankel, Kind and King, Mally and Evelyn, and Lowry et al., respectively.[17-20]
Estimation of in vivo antioxidant markers
Measurement of antioxidant enzymes CAT, SOD, and GPx was done using the methods described by Aebi, Beauchamp and Fridovich, and Rotruck et al., respectively.[21-23] The peroxidation level was estimated in terms of TBARS in liver, and reduced glutathione was measured by the method of Ellman.[24]
Histopathological studies
The liver tissues fixed in 10% formol saline for 48 h were dehydrated by passing successively in different grades of ethyl alcohol, cleared in xylene and embedded in paraffin. Sections of 4–5 mm thickness were cut, stained with hematoxylin and eosin dye for microscopic observation.
Statistical analysis
Results were expressed as a mean ± standard error of the mean, the statistical analysis was carried out by one-way ANOVA followed by Dunnett’s multiple comparison tests and P < 0.05 was considered as significant.
Results and Discussion
Behavioral and toxicological studies
CHL showed no gross behavioral changes, exerted no toxic effects and mortality on animals at the tested doses up to 2000 mg/kg which indicated CHL to be safe to carry out in vivo studies at the selected doses (100 mg/kg and 200 mg/kg).
Estimation of total phenolic and flavonoid contents
The TPC and TFC of CHL were measured to be 70 ± 14 mg GAE/g and 185 ± 18 mg/g, respectively. Total phenol and flavonoid contents of plant extracts are regarded to be correlated with antioxidant activity as phenolics are proved to be strong antioxidants. CHL was analyzed to contain a good amount of phenols and flavonoids which implicated its usefulness as a potential antioxidant and hepatoprotective agent.
In vitro antioxidant studies
Free radicals are chemical entities with one or more unpaired electrons and can exist separately. Proteins, DNA, and lipids are susceptible to attack by free radicals as their generation can lead to thousands of reactions that can result in tissue damage. Antioxidants play an important role in reducing the free radical damage by scavenging them. The in vitro antioxidant potential of CHL was studied by measuring the ability of CHL to inhibit various free radicals through reducing power assay, inhibition of lipid peroxidation and different free radicals scavenging assays. Reducing power assay is an indicative of the ability of an antioxidant to donate an electron which is an important mechanism of phenolic antioxidant action.[25] Reducing power is often correlated to the antioxidant activities of plant extracts. In this assay, the ability of CHL to reduce ferric cyanide complex (Fe3+) to ferrous cyanide form (Fe2+) resulting an increase in the absorbance of the reaction mixture was analyzed. The dose-dependent reductive capability of CHL is shown in Figure 1a. CHL showed an increase in reductive ability with increase in concentration as evident from the increase in absorbance of the reaction mixture. CHL exhibited a EC50 value of 320 μg/ml compared with BHA which showed a EC50 value of 310 μg/ml. The study indicated that CHL could act as an electron donor and may react with free radicals to terminate free radical chain reactions. The reductive ability of CHL may be responsible for its antioxidant activity. Lipid peroxidation leads to the formation of lipid radicals through oxidative degradation of polyunsaturated fatty acids present in brain, liver, and causes cell injury.[26] CHL exerted a concentration-dependent inhibition of lipid peroxidation in the tested concentration range (50–500 μg/ml). The IC50 value of CHL was 230 μg/ml, and that of BHA was 260 μg/ml which indicated a significant inhibition on lipid peroxidation by CHL (Figure 1b). Lipid peroxidation is initiated by ferrous sulfate through OH by Fenton’s reaction.[27] Inhibition of lipid peroxidation by CHL may be due to the scavenging of OH or minimizing the rate of conversion of ferrous to ferric or by chelating with iron. DPPH was used to evaluate the proton radical scavenging ability of CHL as it possesses a proton free radical. The scavenging effect of CHL on DPPH radical is given in Figure 1c. The IC50 value obtained for DPPH scavenging effect of CHL was 320 μg/ml and that for BHA was 400 μg/ml. The results demonstrated CHL to be as more potent than BHA in DPPH radical scavenging activity. Superoxide anion radicals are produced by oxidative enzymes from molecular oxygen and through auto-oxidation of catecholamines.[28,29] The assay was based on the inhibition of superoxides which reduce NBT to a blue colored formation. Superoxide anion radical scavenging activity of CHL is given in Figure 1d. The results indicate CHL to be more efficient in scavenging superoxide anion radicals generated in vitro in a concentration-dependent manner. IC50 value of CHL was 280 μg/ml, and that of BHA was 350 μg/ml. OH inhibition was estimated by the ability of CHL to scavenge the OHs generated by Fe3+/ascorbate/EDTA/H2O2 through Fenton’s reaction. As given in Figure 1e, CHL and BHA both exhibited increased OH inhibition with increase in concentration. CHL showed potent OH scavenging ability than the standard as manifested by the IC50 values, 290 μg/ml for CHL and 360 μg/ml for BHA. In vitro, antioxidant studies clearly demonstrated the significant antioxidant and free radical scavenging activities of CHL which further encouraged us to do in vivo evaluation.
In vivo studies
Antioxidant and hepatoprotective potential of CHL was evaluated using PCM-induced hepatotoxicity in rats. Administration of PCM (1 g/kg) caused a significant liver damage as evidenced from the increase in serum levels of GOT, GPT, ALP, and BRN to 856.12 ± 11.8 U/l, 216.95 ± 14.8 U/l, 318.61 ± 8.16 U/l, and 5.83 ± 0.91 mmol/l in PCM control group compared to the values of normal control group which showed 205.28 ± 15.7 U/l, 66.16 ± 4.15 U/l, 170.51 ± 11.6 U/l, and 2.44 ± 0.17 mmol/l, respectively (Table 1). The increased levels of hepatic biochemical markers in PCM intoxicated animals were significantly (P < 0.05, P < 0.01) reduced by CHL treated (100 mg/kg, 200 mg/kg). TP level was lowered in PCM intoxicated group at 51.67 ± 2.17 mmol/l, and it was increased in CHL pretreated (200 mg/kg) group to near normalcy. PCM is a safe antipyretic and analgesic agent at therapeutic doses, but overdose can cause fatal hepatic necrosis in experimental animals and humans.[30,31] Paracetamol at high doses produces liver necrosis due to its bio-activation to a toxic electrophile, N-acetyl-p-benzoquinone-imine (NAPQI) by cytochrome P450 monooxygenase.[32] Hepatotoxic agents cause hepatic injury which leads to leaking of hepatocellular enzymes into the plasma due to the defect caused in the transport function of hepatocytes.[33] The measurement of serum enzymes levels is a useful quantitative marker to know the extent of hepatic damage due to hepatotoxic agents. In this present study, the rats intoxicated with PCM showed an increase in serum levels of hepatic enzymes which confirmed the hepatic damage. CHL pretreatment (100mg/kg, 200mg/kg) resulted in significant reduction (P < 0.05, P < 0.01) in serum levels of hepatic enzymes GOT, GPT, and ALP and may have exerted a protective effect in reducing the liver injury caused by PCM intoxication. This may be due to the inhibitory effects on cytochrome P450 monooxygenase or/ and promotion of its glucuronidation.[34] Suppression of increased ALP activity and reduction of raised bilirubin with CHL treated indicated the stability of biliary dysfunction in PCM intoxicated rat liver.[35] PCM intoxication also caused a decrease (P < 0.001) in serum levels of CAT, SOD, and GPx and glutathione at 91.71 ± 15.62 U/mg protein, 37.32 ± 2.11 U/mg protein, 41.67 ± 2.19 U/mg protein and 19.86 ± 2.13 U/mg protein, respectively, in PCM control group compared to the values of normal control group which showed 276.12 ±7.16 U/mg protein, 90.41 ± 3.61 U/mg protein, 115.07 ± 1.16 U/mg protein and 41.67 ± 1.29 U/mg protein, respectively (Table 2). TBARS level (4.19 ± 0.91nmol/mg protein) was increased (P < 0.001) in PCM -intoxicated group than normal group (2.28 ± 0.81 nmol/mg protein). CHL treatment (100 mg/kg and 200 mg/kg) exhibited a strong counteraction to the hepatotoxic effects of PCM by significantly (P < 0.05 and P < 0.01) increasing the serum levels of CAT (150.19 ± 2.15 U/mg protein), SOD (57.12 ± 2.96 U/mg protein), GPx (75.31 ± 1.29 U/mg protein), glutathione (24.18 ± 1.28 U/mg protein), and decreasing the TBARS level (3.42 ± 0.24 nmol/mg protein) in CHL treated group rats. PCM-induced hepatotoxicity caused a significant reduction (P < 0.001) in glutathione level compared to normal control group. This may be due to the irreversible conjugation of NAPQI with the sulfhydryl groups of glutathione. Glutathione plays an important protective role against free radicals by combining with them to eliminate free radical toxicity.[36] NAPQI mediated depletion of GSH level leads to disruption of calcium homeostasis, mitochondrial dysfunction, and oxidative stress. This oxidative stress results in the engagement and overwhelming of hepatic antioxidant enzymes, SOD, CAT, and GPx by free radicals as evident from decreased levels of these enzymes in PCM intoxicated rat liver. Pre-treatment with CHL increased the levels of SOD, CAT, GPx, and glutathione in CHL pre-treated group. PCMinduced oxidative stress further leads to lipid peroxidation due to the interaction of free radicals with unsaturated lipid CHL brane and releases MDA which is measured in terms of TBARS. A significant increase (P < 0.001) in liver TBARS was observed in PCM control group compared to normal group, and CHL treated reversed the TBARS level in liver. The reversal of hepatic antioxidant defense systems by CHL may be due to the free radical scavenging effect of CHL as supported by in vitro studies. The free radical scavenging effect of CHL may have reversed the NAPQI mediated depletion of glutathione thereby abolished the oxidative stress and lipid peroxidation caused during PCM intoxication. In vivo antioxidant and hepatoprotective activities of CHL were appraised to be dose-dependent as the reversal of serum and hepatic enzymes levels, BRN, glutathione, and TBARS were significantly higher in 200 mg/kg CHL pretreated rats than rats treated with 100 mg/kg of CHL. Moreover, CHL exerted more potent hepatoprotective effect than silymarin (50 mg/kg and 100 mg/kg). Histopathological studies also proved the potent hepatoprotective effect of CHL as given in Figure 2. Microscopic examination of stained liver tissue of normal control rats (Figure 2a) showed normal architecture with hepatic cords, plates radiating from the central vein and with few normal scattered Kupffer cells. Liver sections of PCM intoxicated rats (Figure 2b) exhibited centrilobular necrosis, micro, and macrovesicular fatty changes and scattered lymphomononuclear infiltrate in hepatic parenchyma. CHL treated rats (Figure 2e and f) liver tissues showed a reversal of the liver damage exerted by PCM with few foci of necrosis of hepatocytes and fatty changes and signs of regeneration. The reversal of hepatic damage was found to be higher with 200 mg/kg of CHL administration and was comparable to the standard (Figure 2c and d). In this present study, we report the in vitro and in vivo antioxidant and hepatoprotective activities of CHL for the 1st time. The reported activities of CHL may be due to the presence of phenols and flavonoids as these compounds are proved to be responsible for the antioxidant and hepatoprotective effect of many plants.[37,38] However, further studies on the isolation of the bioactive compounds are needed to identify the bioactive compounds.
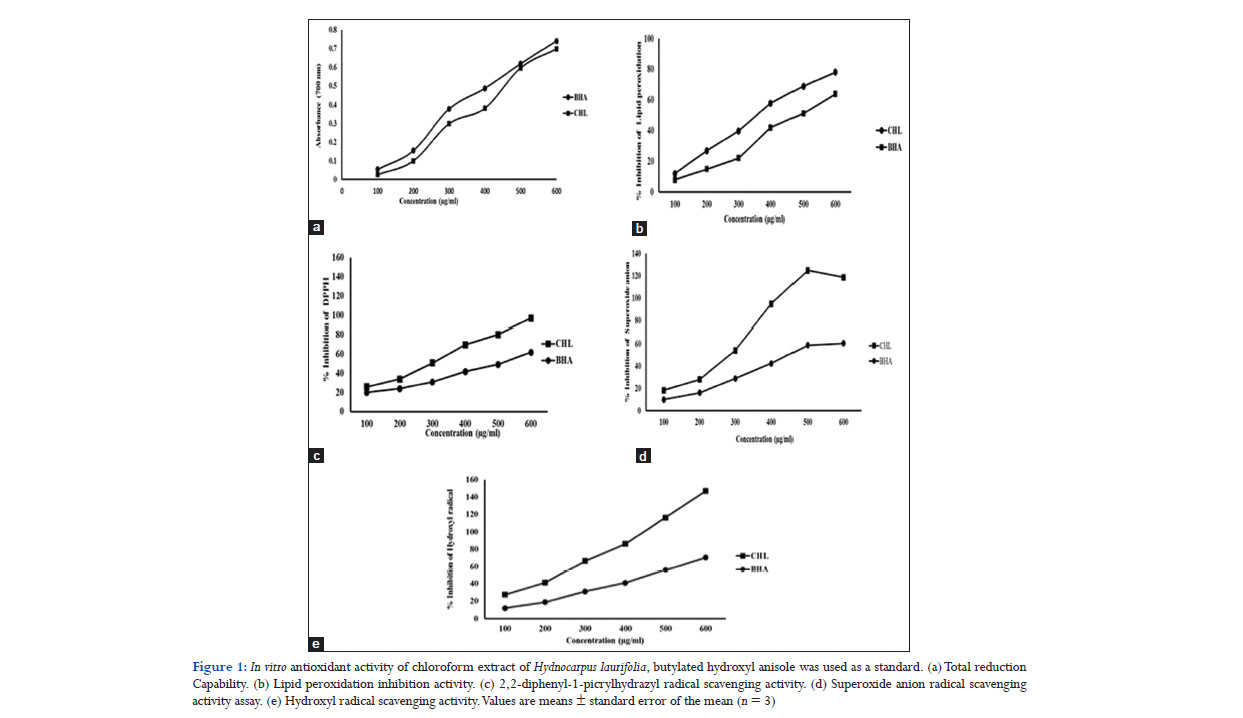
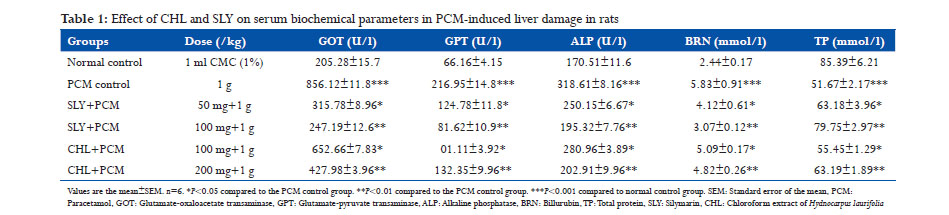
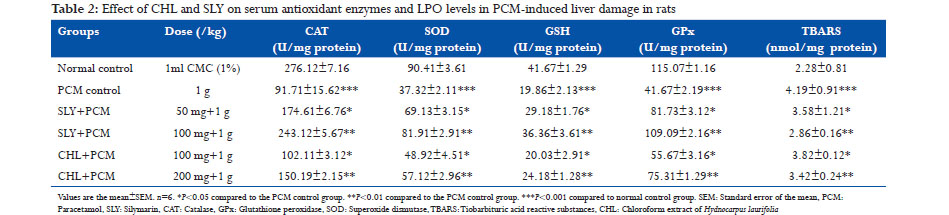
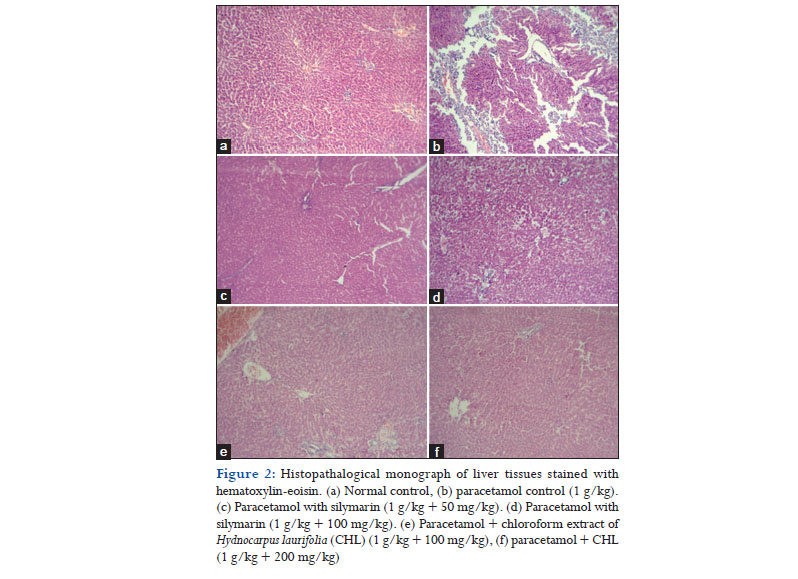
Conclusion
The results obtained in this study demonstrate that the CHL solid antioxidant and hepatoprotective activities mainly due to the induction of antioxidant enzymes and scavenging of reactive free radicals. Further studies are required to elucidate the precise mechanism (s) of antioxidant and hepatoprotective action of HL. The outcome of the study endorsed the traditional use of this plant to treat liver diseases by the inhabitants of Western Ghats of India. Studies are in progress, in our laboratory, to isolate the bioactive compounds responsible for the antioxidant and hepatoprotective activities of HL.
Acknowledgments
We sincerely acknowledge the help extended by Mr. Harihara Sivakumar of KMCH College, of pharmacy during in vivo studies.
References
- Ghani A. Medicinal Plants of Bangladesh: Chemical Constituents and Uses. Dhaka: Asiatic Society of Bangladesh; 1998. p. 467.
- Nadkarni KM. The Indian Materia Medica. Vol. 1. Mumbai: Popular Praksham Pvt. Ltd.; 1979. p. 1153-54.
- Oommen ST, Rao M, Raju CV. Effect of oil of hydnocarpus on wound healing. Int J Lepr Mycobact Dis 1999;67:154.
- Bokov A, Chaudhuri A, Richardson A. The role of oxidative damage and stress in aging. Mech Ageing Dev 2004;125:811-26.
- Giordano FJ, Oxygen, oxidative stress, hypoxia, and heart failure. J Clin Invest 2005;115:500-8.
- Jaeschke H. Reactive oxygen and mechanisms of inflammatory liver injury. J Gastroenterol Hepatol 2000;15:718-24.
- Klaunig JE, Kamendulis LM. The role of oxidative stress in carcinogenesis. Annu Rev Pharmacol Toxicol 2004;44:239-67.
- Rolo AP, Palmeira CM. Diabetes and mitochondrial function: Role of hyperglycemia and oxidative stress. Toxicol Appl Pharmacol 2006;212:167-78.
- Singleton VL, Orthofer R, Lamuela-Raventos RM. Analysis of total phenols and other oxidation substrates and antioxidants by means of folin-ciocalteu reagent. Methods Enzymol 1999;299:152-78.
- Chang C, Yang M, Wen H, Chen J. Estimation of total flavonoids content in propolis by two complementary colorimetric methods. J Food Drug Anal 2002;10:178-82.
- Oyaizu M. Studies on products of browning reaction: Antioxidant activities of products of browning reaction prepared from glucosamine. J Nutr 1986;44:307-15.
- Ohkawa H, Ohishi N, Yagi K. Assay for lipid peroxide in animal tissue by thiobarbituric acid reaction. Anal Biochem 1979;95:351-58.
- Kumar VP, Shasidhara S, Kumar MM, Sridhara BY. Effect of Luffa echinata on lipid peroxidation and free radical scavenging activity. J Pharm Pharmacol 2000;52:891-94.
- Blois MS. Antioxdant determinations by the use of a stable free radical. Nature 1958;181:1199-200.
- Nishikimi M, Rao NA, Yagi K. The occurrence of superoxide anion in the reaction of reduced phenazine methosulfate and molecular oxygen. Biochem Biophys Res Commun 1972;46:849-54.
- Aruoma OI, Halliwell B. Action of hypchlorous acid on the antioxidant protective enzymes superoxide dismutase, catalase and glutathione peroxidise. Biochem J 1987;248:973-76.
- Reitman S, Frankle S. A colorimetric method for the determination of serum glutamic oxaloacetic and glutamic pyruvic transaminase. Am J Clin Pathol 1957;28:56-63.
- Kind PR, King D. In vitro determination of serum alkaline phosphatase. J Clin Pathol 1972;7:321-22.
- Mally HT, Evelyn KA. Estimation of serum bilirubin level. J Biol Chem 1937;191:481-90.
- Lowry OH, Rosebrough NJ, Farr AL, Randall RJ. Protein measurement with the folin phenol reagent. J Biol Chem 1951;193:265-75.
- Aebi H. Catalase in vitro. Methods Enzymol 1984;105:121-6.
- Beauchamp C, Fridovich I. Suoeroxide dismutase: Improved assays and an assay applicable to acrylamide gels. Anal Biochem 1971;44:276-87.
- Rotruck JT, Pope AL, Gather HE, Swason AB, Hafeman DG, Hoekstra WG. Selenium: Biochemical role as acomponent of glutathione peroxidise. Science 1973;179:588-90.
- Ellman Gl. Tissue sulphydryl groups. Arch Biochem Biophys 1959;82:70-7.
- Mohamed H, Ons M, Yosra ET, Rayda S, Neji G, Moncef N. Chemical composition and antioxidant and radical-scavenging activities of Periploca leavigata root bark extracts. J Sci Food Agric 2009;89:897-905.
- Coyle JT, Puttfarcken P. Oxidative stress, glutamate, and neurodegenerative disorders. Science 1993;262:689-95.
- Braughler JM, Duncan LA, Chase RL. The involment of iron in lipid peroxidation. Importance of ferric to ferrous reactions in initiation. J Biol Chem 1986;261:10282-9.
- Sainani GS, Manika JS, Sainani RG. Oxidative stress: A key factor in pathogenesis of chronic diseases. Med Update 1997;1:1-4.
- Hemnani T. Parihar MS. Reactive oxygen species and oxidative DNA damage. Indian J Physiol Pharmacol 1998;42:440-52.
- Amar PJ, Schiff ER. Acetaminophen safety and hepatotoxicity--where do we go from here? Expert Opin Drug Saf 2007;6:341-55.
- Schidt FV, Roching FV, Cassey DL, Lee WM. Acetaminophen toxicity in an urban country hospital. N Engl J Med 1997;337:1112-17.
- Dahlin DC, Miwa, GT, Lu AY, Nelson SD. N-acetyl-p-benzoquinone imine: A cytochrome P-450-mediated oxidation product of acetaminophen. Proc Natl Acad Sci U S A 1984;81:1327-31.
- Zimmerman HJ, Seeff LB. Enzymes in hepatic disease. In: Goodly EL, editor. Diagnostic Enzymology. Philadelphia, PA, USA: Lea & Febiger; 1970. p. 1-38.
- Gilman AG, Rall TW, Nies AS, Taylor P. The Pharmacological Basis of Therapeutics. 13th ed. London: McGraw Hill International Edition; 1992.
- Mukherjee PK. Quality Control of Herbal Drugs. New Delhi: Business Horizons Pharmaceutical Publication; 2002. p. 531.
- Vane JR, Mitchell JA, Appleton I, Tomlinson A, Bishop-Bailey D, Croxtall J. Inducible isoforms of cyclooxygenase and nitric oxide synthase in inflammation. Proc Natl Acad Sci U S A 1994;91:2046-50.
- Oh H, Kim DH, Cho JH, Kim YC. Hepatoprotective and free radical scavenging activities of phenolic petrosins and flavonoids isolated from Equisetum arvense. J Ethnopharmacol 2004;95:421-24.
- Sharda B. Free radicals: Newer aspects. In: Vohora SB, Agarwal VP, editors. Toxicological and Environmental Health. New Delhi: Asia Tech Publications Inc.; 1999. p. 97.
|